Quantinuum’s H-Series team has hit the ground running in 2023, achieving a new performance milestone. The H1-1 trapped ion quantum computer has achieved a Quantum Volume (QV) of 32,768 (215), the highest in the industry to date.
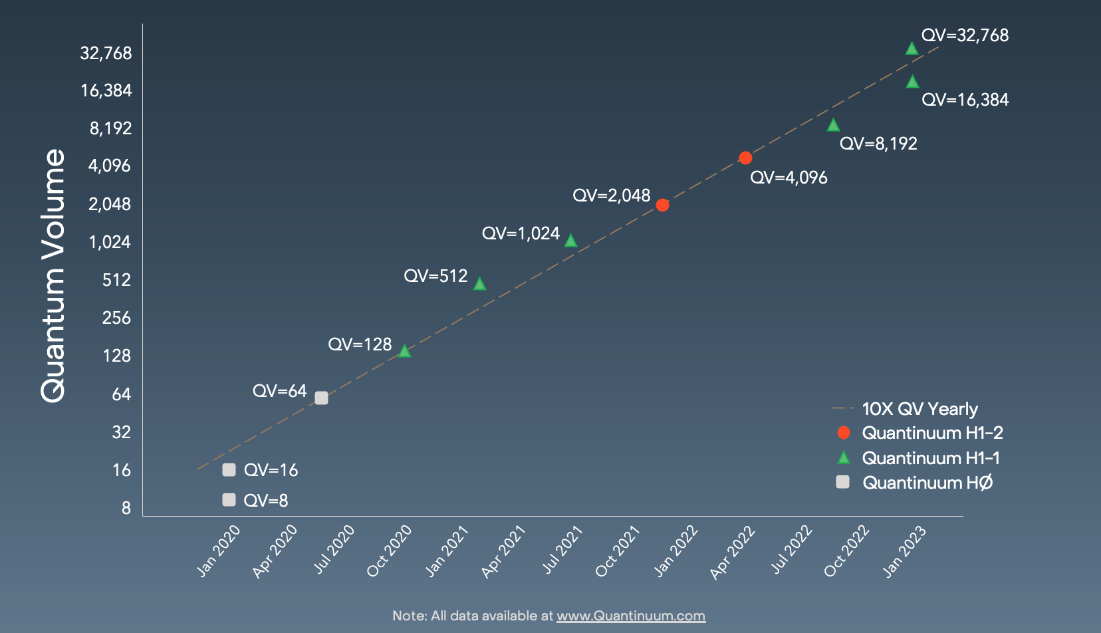
The team previously increased the QV to 8,192 (or 213) for the System Model H1 system in September, less than six months ago. The next goal was a QV of 16,384 (214). However, continuous improvements to the H1-1's controls and subsystems advanced the system enough to successfully reach 214 as expected, and then to go one major step further, and reach a QV of 215.
The Quantum Volume test is a full-system benchmark that produces a single-number measure of a quantum computer’s general capability. The benchmark takes into account qubit number, fidelity, connectivity, and other quantities important in building useful devices.1 While other measures such as gate fidelity and qubit count are significant and worth tracking, neither is as comprehensive as Quantum Volume which better represents the operational ability of a quantum computer.
Dr. Brian Neyenhuis, Director of Commercial Operations, credits reductions in the phase noise of the computer’s lasers as one key factor in the increase.
"We've had enough qubits for a while, but we've been continually pushing on reducing the error in our quantum operations, specifically the two-qubit gate error, to allow us to do these Quantum Volume measurements,” he said.
The Quantinuum team improved memory error and elements of the calibration process as well.
“It was a lot of little things that got us to the point where our two-qubit gate error and our memory error are both low enough that we can pass these Quantum Volume circuit tests,” he said.
The work of increasing Quantum Volume means improving all the subsystems and subcomponents of the machine individually and simultaneously, while ensuring all the systems continue to work well together. Such a complex task takes a high degree of orchestration across the Quantinuum team, with the benefits of the work passed on to H-Series users.
To illustrate what this 5-digit Quantum Volume milestone means for the H-Series, here are 5 perspectives that reflect Quantinuum teams and H-Series users.
Perspective #1: How a higher QV impacts algorithms
Dr. Henrik Dreyer is Managing Director and Scientific Lead at Quantinuum’s office in Munich, Germany. In the context of his work, an improvement in Quantum Volume is important as it relates to gate fidelity.
“As application developers, the signal-to-noise ratio is what we're interested in,” Henrik said. “If the signal is small, I might run the circuits 10 times and only get one good shot. To recover the signal, I have to do a lot more shots and throw most of them away. Every shot takes time."
“The signal-to-noise ratio is sensitive to the gate fidelity. If you increase the gate fidelity by a little bit, the runtime of a given algorithm may go down drastically,” he said. “For a typical circuit, as the plot shows, even a relatively modest 0.16 percentage point improvement in fidelity, could mean that it runs in less than half the time.”
To demonstrate this point, the Quantinuum team has been benchmarking the System Model H1 performance on circuits relevant for near-term applications. The graph below shows repeated benchmarking of the runtime of these circuits before and after the recent improvement in gate fidelity. The result of this moderate change in fidelity is a 3x change in runtime. The runtimes calculated below are based on the number of shots required to obtain accurate results from the benchmarking circuit – the example uses 430 arbitrary-angle two-qubit gates and an accuracy of 3%.

Perspective #2: Advancing quantum error correction
Dr. Natalie Brown and Dr, Ciaran Ryan-Anderson both work on quantum error correction at Quantinuum. They see the QV advance as an overall boost to this work.
“Hitting a Quantum Volume number like this means that you have low error rates, a lot of qubits, and very long circuits,” Natalie said. “And all three of those are wonderful things for quantum error correction. A higher Quantum Volume most certainly means we will be able to run quantum error correction better. Error correction is a critical ingredient to large-scale quantum computing. The earlier we can start exploring error correction on today’s small-scale hardware, the faster we’ll be able to demonstrate it at large-scale.”
Ciaran said that H1-1's low error rates allow scientists to make error correction better and start to explore decoding options.
“If you can have really low error rates, you can apply a lot of quantum operations, known as gates,” Ciaran said. "This makes quantum error correction easier because we can suppress the noise even further and potentially use fewer resources to do it, compared to other devices.”
Perspective #3: Meeting a high benchmark
“This accomplishment shows that gate improvements are getting translated to full-system circuits,” said Dr. Charlie Baldwin, a research scientist at Quantinuum.
Charlie specializes in quantum computing performance benchmarks, conducting research with the Quantum Economic Development Consortium (QED-C).
“Other benchmarking tests use easier circuits or incorporate other options like post-processing data. This can make it more difficult to determine what part improved,” he said. “With Quantum Volume, it’s clear that the performance improvements are from the hardware, which are the hardest and most significant improvements to make.”
“Quantum Volume is a well-established test. You really can’t cheat it,” said Charlie.
Perspective #4: Implications for quantum applications
Dr. Ross Duncan, Head of Quantum Software, sees Quantum Volume measurements as a good way to show overall progress in the process of building a quantum computer.
“Quantum Volume has merit, compared to any other measure, because it gives a clear answer,” he said.
“This latest increase reveals the extent of combined improvements in the hardware in recent months and means researchers and developers can expect to run deeper circuits with greater success.”
Perspective #5: H-Series users
Quantinuum’s business model is unique in that the H-Series systems are continuously upgraded through their product lifecycle. For users, this means they continually and immediately get access to the latest breakthroughs in performance. The reported improvements were not done on an internal testbed, but rather implemented on the H1-1 system which is commercially available and used extensively by users around the world.
“As soon as the improvements were implemented, users were benefiting from them,” said Dr. Jenni Strabley, Sr. Director of Offering Management. “We take our Quantum Volume measurement intermixed with customers’ jobs, so we know that the improvements we’re seeing are also being seen by our customers.”
Jenni went on to say, “Continuously delivering increasingly better performance shows our commitment to our customers’ success with these early small-scale quantum computers as well as our commitment to accuracy and transparency. That’s how we accelerate quantum computing.”
Supporting data from Quantinuum’s 215 QV milestone
This latest QV milestone demonstrates how the Quantinuum team continues to boost the performance of the System Model H1, making improvements to the two-qubit gate fidelity while maintaining high single-qubit fidelity, high SPAM fidelity, and low cross-talk.
The average single-qubit gate fidelity for these milestones was 99.9955(8)%, the average two-qubit gate fidelity was 99.795(7)% with fully connected qubits, and state preparation and measurement fidelity was 99.69(4)%.
For both tests, the Quantinuum team ran 100 circuits with 200 shots each, using standard QV optimization techniques to yield an average of 219.02 arbitrary angle two-qubit gates per circuit on the 214 test, and 244.26 arbitrary angle two-qubit gates per circuit on the 215 test.
The Quantinuum H1-1 successfully passed the quantum volume 16,384 benchmark, outputting heavy outcomes 69.88% of the time, and passed the 32,768 benchmark, outputting heavy outcomes 69.075% of the time. The heavy output frequency is a simple measure of how well the measured outputs from the quantum computer match the results from an ideal simulation. Both results are above the two-thirds passing threshold with high confidence. More details on the Quantum Volume test can be found here.
Heavy output frequency for H1-1 at 215 (QV 32,768)

Heavy output frequency for H1-1 at 214 (QV 16,384)

Quantum Volume data and analysis code can be accessed on Quantinuum’s GitHub repository for quantum volume data. Contemporary benchmarking data can be accessed at Quantinuum’s GitHub repository for hardware specifications.